At its March meeting, the Board of Regents of the Nevada System of Higher Education recognized the contributions of rising and established researchers across its eight institutions. Foundation Professor Ian Buckle was named the Regents’ Distinguished Researcher, honoring an exemplary career that has advanced seismic engineering nationally and globally in innumerable ways. While serving as the CCEER director, Buckle raised funds for the construction of the Earthquake Engineering Laboratory. Opened in 2014, this Laboratory simultaneously doubled the University’s capacity to conduct large-scale experiments in earthquake engineering, and propelled its earthquake engineering research program to international renown, making it among the largest and most prestigious in the world.
“It is an honor to receive this award, not just for me but for the earthquake engineering program at UNR,” Buckle said. “Tweaking Isaac Newton just a little bit: If I have been able to go far, it is because I am standing on the shoulders of giants… those who preceded me in this program and those who have had my back the last two decades. From academic colleagues to lab and admin staff across campus, too many to name, I am deeply indebted. Success winning such a competitive award is a win for the entire team, including my graduate students. I am proud of each and every one of them.”
College of Engineering Dean Manos Maragakis said of the award, “This a very well-deserved award. Dr. Buckle’s hard work, deep and broad knowledge of the field, dedication and work ethic have made a big impact on earthquake engineering worldwide and a long-lasting, transformative impact on our program, the College and the University.”
Highlights in a distinguished career
Base Isolation of the City and County Building in Salt Lake City
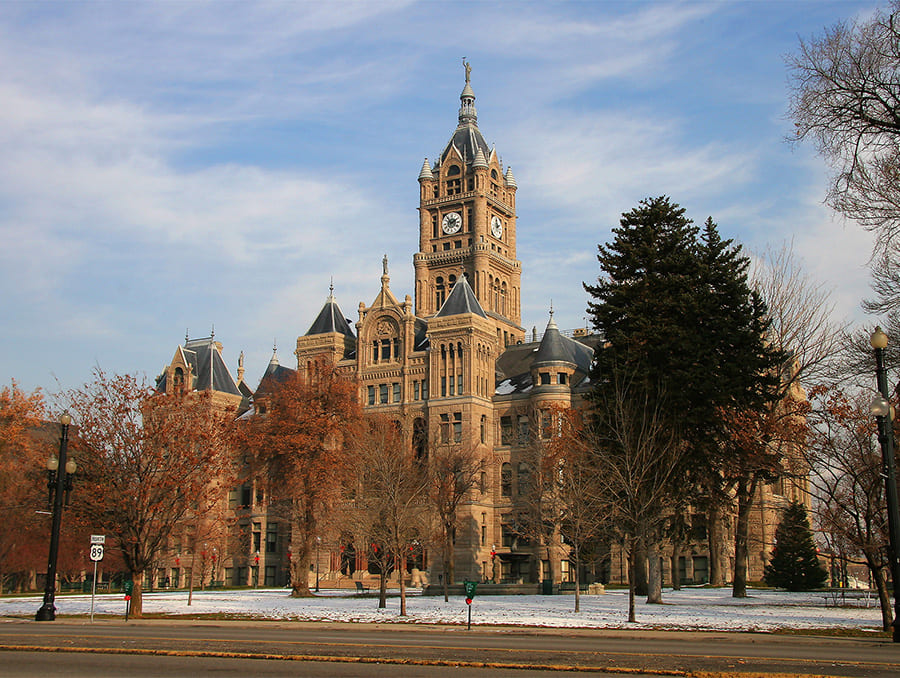
After receiving his Ph.D. in bridge engineering from the University of Auckland, Buckle was attracted to a burgeoning new field in earthquake engineering: base isolation, in which a buffer is created between a structure (building, bridge or industrial plant) and the ground beneath it. If designed correctly, this buffer can dramatically reduce the damaging impact of large earthquakes on the structure.
“Base isolation separated a building from the ground by putting it on flexible supports in its basement. Now it’s called ‘seismic isolation’- not all structures have basements, after all. At the time, I was one of only a handful of people worldwide who were excited by the potential of this new approach to earthquake engineering.”
Buckle was able to put into practice the seismic isolation techniques he’d been researching in 1984 when an historic structure in Salt Lake City was threatened with demolition. Originally opened in 1894 to replace the Salt Lake City Council Hall and the Salt Lake County Courthouse, the regal City and County Building was up for demolition and replacement because it was a life-safety hazard in the event of a strong earthquake.
“Salt Lake City is seismically active, and the City and County Building is an unreinforced stone building,” Buckle said. “Such masonry does not do well in cold weather or an earthquake.”
On the one hand, masonry deteriorates in freeze-thaw cycles over time, while on the other hand, structures of the vintage of the City and County Building were not seismically designed and are particularly vulnerable to earthquakes. They are unreinforced and every earthquake threatens their integrity endangering anyone in or near the building. Because of its importance to the community and its historical and aesthetic value, avoiding the scrapheap was paramount. Buckle joined a team to isolate the building to reduce the earthquake forces in the fragile masonry. It became one of the first buildings in the U.S. to be retrofitted using isolation. He designed the isolators, supervised their installation and, by doing so, helped keep the building from demolition. Today it continues to serve the community as a municipal building.
Not long afterward, the Mackay Mines Building on campus was seismically isolated in a similar way, so when Buckle came to the University, he began taking his students to the latter in order to show them a real-world application of one of the most innovative technologies in structural engineering today.
Shake tables
When Buckle arrived at the University in 1999, the University had two large “shake tables.” These tables allowed researchers to simulate earthquakes in the laboratory to test new materials and construction techniques for bridges and other structures using almost life-size models. At the time, the two shake tables only moved in one direction.
“An opportunity arose to enhance our facility by adding a third table and make them all biaxial,” Buckle said, referring to the ability to shake the tables both east-west and north-south, increasing their experimental possibilities. “We had funds left over at the end of the process, and the sponsor encouraged us to put it good use rather than return it. So we built a fourth table. Having four large tables was unique in the United States, if not the world.”
With such an array of shake tables, Buckle was immediately able to perform experiments that could not have been done before. Specifically, he built an almost half-scale model of a curved bridge that spanned all four shake tables. In a widely reported experiment, he placed six trucks on the bridge to see what effect, if any, the trucks would have on the performance of the bridge during an earthquake.
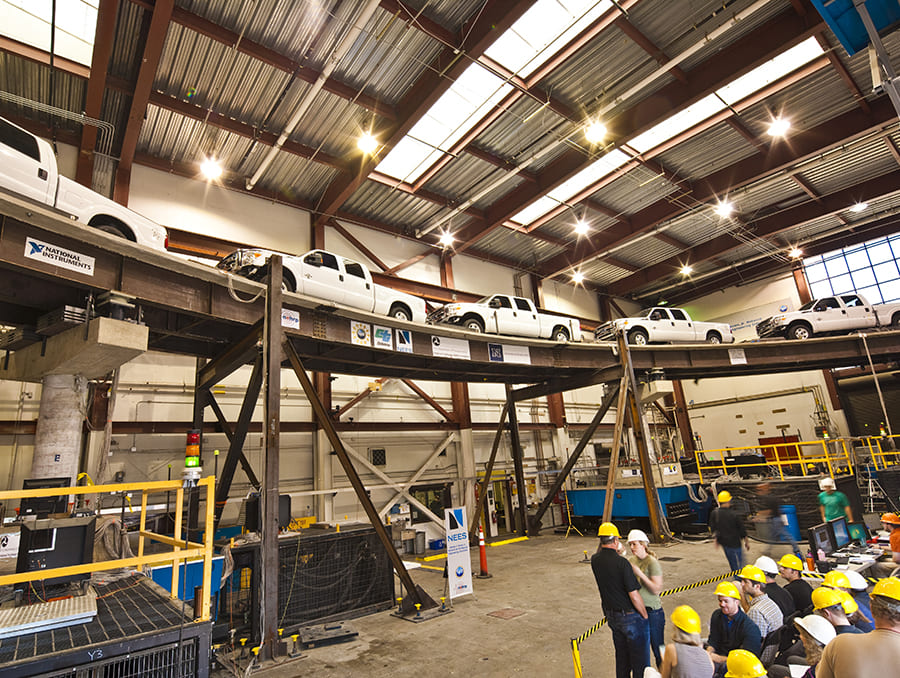
“At the time bridges were not designed to withstand a major earthquake while fully loaded with trucks because it was very unlikely both would occur at the same time.” Buckle explained. “But Caltrans was concerned that with increasing truck traffic on its freeways and especially near large ports, this assumption may no longer be true and wanted to know whether the presence of trucks made a bridge more vulnerable during an earthquake due to the extra weight.
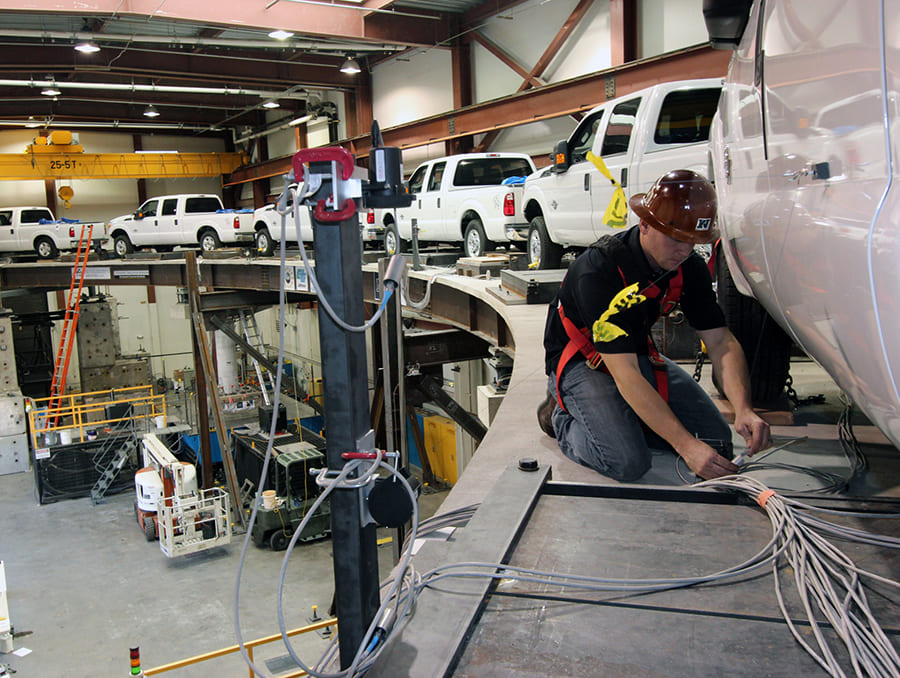
“So we were given additional funding to put trucks on the bridge,” Buckle said. “And it behaved better with the vehicles in place than without. Turns out the suspension systems in the individual trucks helped the performance of the bridge, in a manner similar to the way a tuned mass damper controls wind vibrations in tall buildings.”
While answering an interesting question about the relationship between a bridge and the load it carries, the experiment put Caltrans at ease. The upshot of the results was that they need not be concerned about the simultaneous occurrence of an earthquake and nose-to-tail truck traffic.
Earthquake Engineering Laboratory: “earthquakes on demand”
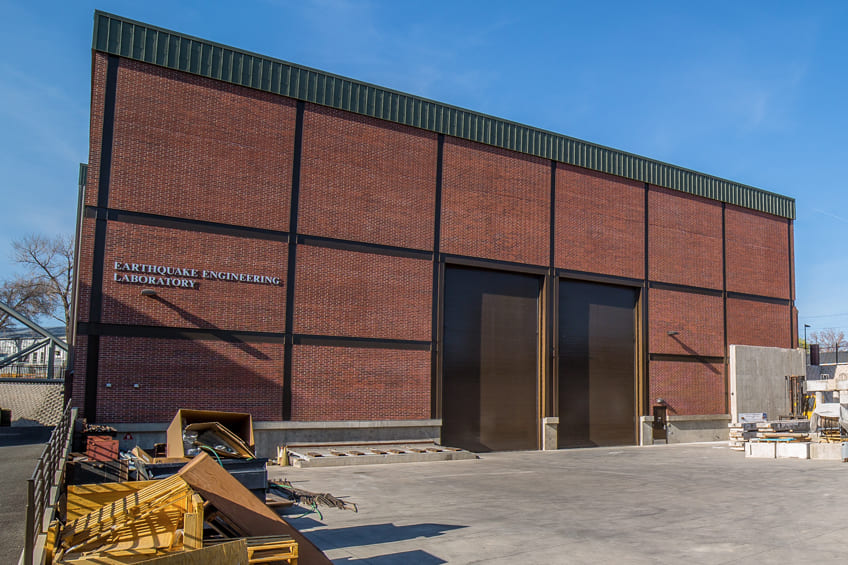
With the growing success of the earthquake engineering program, Buckle began fundraising for a new building dedicated to earthquake engineering with additional floor space for the shake tables. After raising 80 percent of the funding through federal sources and receiving a 20 percent cost-share from the University, the Earthquake Engineering Laboratory Building (EEL) became a reality in 2014. Along the way, Buckle and the rest of CCEER had to make strategic choices about how to configure the new space.
“Since we are starting with a clean slate, how can we build a world-class laboratory given the budget constraints?” Buckle said. “When you buy a house built by someone else, you might complain about the layout—why is the laundry on this side of the house and not the other? But when you build your own house, such complaints fall on deaf ears.”
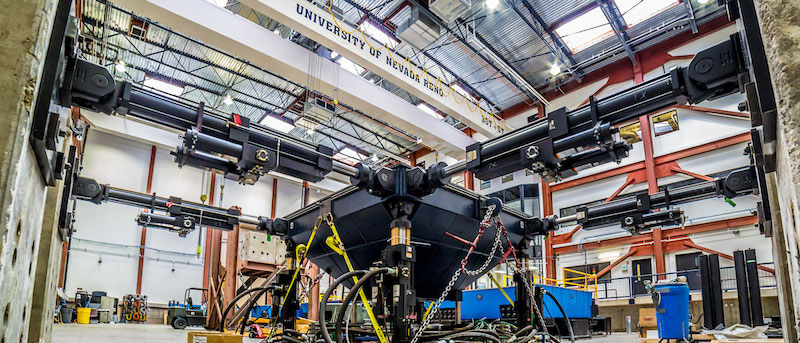
After an exhaustive planning process, the building was designed to accommodate the various needs of the program. The third level houses graduate students, the middle level has office space for professional staff, visitors and a conference room, and the first level houses the control room, offices for lab staff, a tool room, a transducer calibration room, and a server room. In addition to an auditorium for workshops, conferences, and seminars, the first floor also includes the crowning jewel of the building: the high-bay laboratory, which houses the shake table array, where experiments with international implications are conducted by students and faculty alike.
“Fortunately, damaging earthquakes are rare,” Buckle said. “This fact is good for society, but not so good for studying the effects of earthquakes on the structures we build. Our Laboratory addresses this problem. We can have earthquakes on demand and test systems with innovative details. If someone has a bright idea today, we can test it tomorrow. And not have to wait fifty, maybe a hundred years, to find out it was not such a good idea.”
Soil box
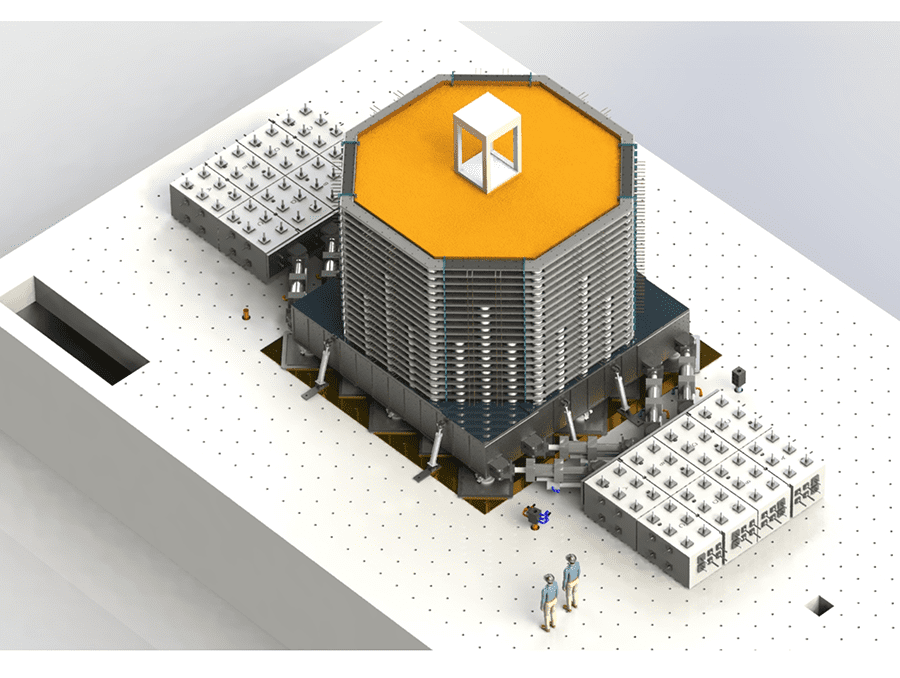
With the original four shake tables moved into the EEL, space was created in the James E. Rogers and Louis Wiener Jr. Large-Scale Structures Lab for a new project. Still in development, the so-called “soil box” is a biaxial, 19-layer, laminar box designed to address both halves of the equation in earthquake engineering.
“Traditionally, structural engineers work above the ground, ignoring the effect of soil,” Buckle said. “Meanwhile, geotechnical engineers focus on the soil below but ignore the building above. But the building and the soil are in fact one system.”
The behavior of the soil affects the building resting upon it, and the building’s weight affects the soil beneath it. To understand what a bridge, building or other structure would do in an earthquake requires knowing about ‘soil-structure interaction.’
“How do you do this kind of experiment? Soil is heavy, and its properties change with depth due to confinement,” Buckle said. “One way to simulate confined soil is to spin it in a centrifuge, but the size of an embedded structure is very small. Our approach is to build as-big-a-box of soil as we can afford and put it on as-big-a-shake table as we can afford.”
Upon its completion, the soil box will hold 400 tons of soil and be the largest of its kind in the United States. Sponsored by the Department of Energy, and a collaboration between the University, the Lawrence Berkeley National Laboratory in Berkeley, Calif., and the University of California, Davis, the many applications of the box include research to improve the seismic safety of nuclear facilities. As such, it supports the goal of the Department of Energy to secure public safety and represents another avenue for the researchers at the University to undertake ground-breaking research that raises the bar for the field.
Tsunamis and bridges
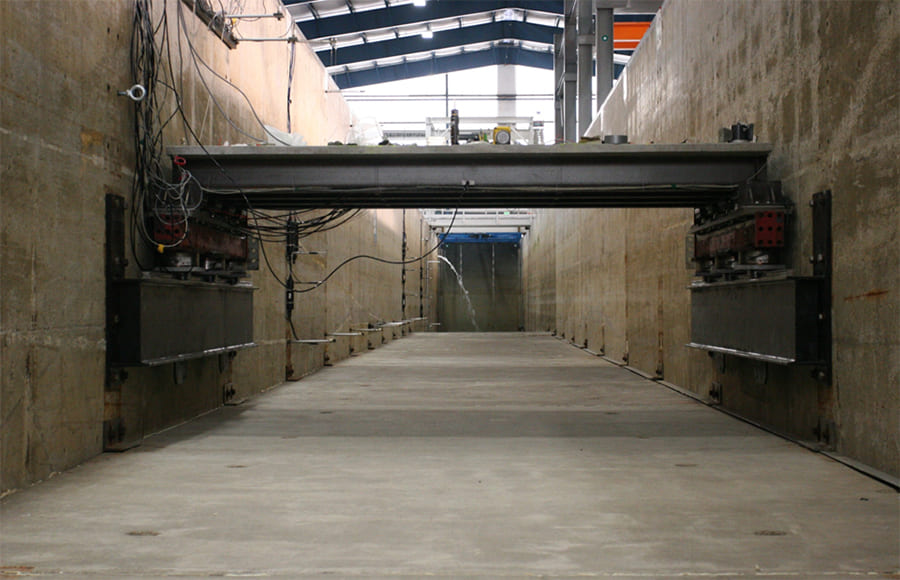
While developing the shake table array at the University over the past decade, Buckle has also been involved in responding to an event in Japan in 2011. On March 11, the Tōhoku Earthquake hit off the coast of Japan. The fourth most powerful earthquake in the world since 1900, it resulted in a tsunami that reached more than 40 meters in height. While much of the world was focused on the resulting failure of the power systems in the Fukushima Daiichi Nuclear Power Plant, Buckle noticed something interesting about the coastal bridges that had been struck by this powerful tsunami.
“When the 2011 tsunami hit the east coast of Japan, about half of the bridges survived. Half did not,” Buckle said. “Why?”
Noting that the forces applied by a tsunami can be so strong it isn’t economically feasible to build a bridge to withstand them, Buckle observed that those bridges that survived the battering had superstructures that were an integral part of the supporting substructure.
With this observation in mind, Buckle developed a research program to better understand the effects of tsunamis on coastal bridges, including those whose superstructures were not integral with their substructures. Working in the Large Wave Flume at Oregon State University in Corvallis, Ore., he placed a large-scale model of a bridge in the flume and subjected it to tsunami-like waves.
Among the challenges facing those designing bridges to survive a tsunami is the combined effect of a large horizontal force and vertical uplift,” Buckle said. “When a tsunami strikes, air trapped underneath a bridge pushes it up violently. Unless specifically designed for this extreme upward push, the girders of these bridges can be lifted off their seats.
“Trapped air under a bridge applies a tremendous uplift load. A solution is to let the air escape quickly by venting the bridge deck. But during rain, holes in the deck of a bridge could drain rainwater with pollutants into the stream below. So, how do you do this and still satisfy environmental concerns? Perhaps install one-way valves in the vents or a catch-basin below the bridge to trap pollutants? Either way the benefit will be the survival of the bridge and its immediate availability for post-event recovery.”
The results of Buckle’s research will provide much-needed guidance to engineers designing new—and retrofitting existing—coastal bridges.
“There has been nothing ‘out there’ to tell engineers what to do. But our results have recently been included in a new guide specification for the design of bridges subject to tsunami inundation.... Seldom does research get into practice so quickly.”
“There has been nothing ‘out there’ to tell engineers what to do. But our results have recently been included in a new guide specification for the design of bridges subject to tsunami inundation. It is expected to go to ballot by the American Association of State Highway and Transportation Officials in July 2021,” Buckle said. “To become a ‘code,’ this specification must be approved by a majority of the 50 State Bridge Engineers in the U.S., who vote once a year on such proposals. Seldom does research get into practice so quickly.”
But Buckle is quick to note that none of the above would have been possible without the skill and hard work of his graduate students and post-doctoral scholars. Too many to list by name, Buckle is indebted to each and every one of them. He is also grateful for the assistance of numerous state and federal agencies who have financially supported his work.
Buckle’s work in the earthquake engineering community includes service as President of the Earthquake Engineering Research Institute, and in 2015, he was a co-recipient of the American Institute of Steel Construction’s Educator Special Achievement Award. In addition, he received the prestigious Martin C. Duke Award in Lifeline Earthquake Engineering from the American Society of Civil Engineers in 2016. This latest award from the NSHE Board of Regents is a fitting acknowledgement of a lifetime of achievements.