Buildings made of mass timber – layers of wood bonded together – are gaining popularity as greener and faster alternatives to concrete and steel structures. With new building codes recently updated to permit more high-rise mass-timber buildings to be constructed in the United States, many have questioned how such buildings would fare in earthquakes.
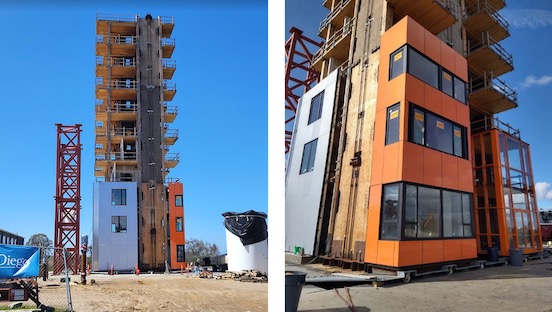
The Natural Hazards Engineering Research Infrastructure (NHERI) TallWood project aims to prove the resilience of tall timber buildings by simulating a series of large earthquakes on a full-scale, 10-story mass timber building at the NHERI@UCSD facility – the world’s tallest full-scale building ever tested on an earthquake shake table. The research project is funded by the U.S. National Science Foundation. The key feature of the building is a post-tensioned rocking wall system built from mass timber panels. Under lateral forces, the wood panels rock up off the base, and the post-tensioning rods re-center the system after the earthquake passes. This new system is aimed at resilient performance, which means the building will have minimal damage from design level shaking and be quickly repairable after rare earthquakes.
The project is led by Professor Shiling Pei from Colorado School of Mines, and the structure was designed and built by a large team of academic and industry collaborators. Professor Keri Ryan of the Civil & Environmental Engineering Department at the University of Nevada, Reno has played a key role on the leadership team.
Non-structural systems research
Ryan and her team coordinated the development and inclusion of all the building’s non-structural systems. “Resilient design must also account for the building’s non-structural systems, which are not part of the structural load-resisting system, but play an important role in the building’s function and its ability to recover after the earthquake,” said Ryan, project co-investigator and engineering professor at the University. Recent earthquakes have shown that in regions with modern seismic engineering, damage and loss of functionality tends to be concentrated on the non-structural systems.
Some of those non-structural systems include interior walls, stairs and elevators, as well as plumbing, and electric and ventilation systems. “Most seismic engineering research to date has focused solely on the lateral resistance of structural components,” Ryan continues. “However, non-structural components are often highly damaged during earthquakes. After the 1994 Northridge Earthquake, about half of the damage cost to buildings was due to non-structural components. This highlighted the need to address vulnerabilities in non-structural systems.”
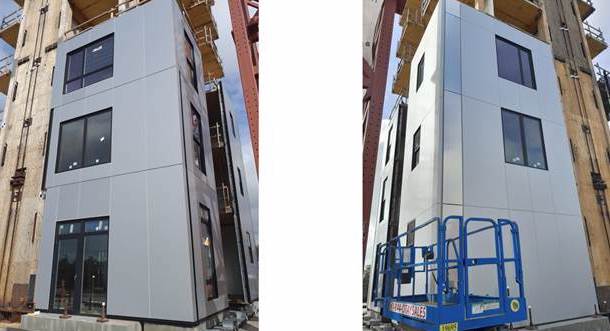
Even if a building remains structurally sound after an earthquake, non-structural damage can ultimately harm its structural integrity. For instance, damage to exterior cladding (the building envelope) can mean that the underlying structure is exposed to weather. Many non-structural components are also crucial to a building’s safety. Damage to stairs, for instance, can trap occupants within a building after a natural disaster, as happened in the 2011 Christchurch Earthquake in New Zealand.
The TallWood project’s walls and stairs
The rocking wall system only works if the other building components can move with it. If the building’s structure is designed to flex during an earthquake, the other building components that are connected between floors need to move with it or risk getting crushed or broken. This is known as deformation compatibility. “We are focusing on interior walls, the building envelope, and stairs as the most integral non-structural components,” Ryan said. “We are working closely with designers and other specialists to identify how to make improvements wherever we can.”
For interior walls, they are investigating an innovative solution known as a ‘slip track’. “This is when the top of the wall is not directly connected to the above floor slab, and thus can move independently or slip relative to the floor above,” Ryan said. This works well until these walls meet at corners, at which point there is the risk of collision and damage. Now the team is working on the finer details of how to minimize damage at the corners. Ryan’s team is also one of the first to extend the slip concept to exterior facades. Featured prominently on the building’s lower levels are four different exterior façade assemblies, each connected to the structure in a different way in an attempt to prevent or minimize damage.
Stairs are also a big area of interest. “Stairs are typically rigidly connected between floors and are often damaged during earthquakes, and are then deemed unsafe for future use,” Ryan explains. “U.S. building regulations have changed to address this, but there’s still a lot of research that needs to be done to get the best design.” Similar to interior walls, the prime solution is to detach the stairs from the floor at one end, so if the floors move independently the stairs are not stretched or compressed between the two.
Time to get shaking
Executing the project has not been easy. Three years ago, Ryan assembled an industry working group that met bi-weekly, and in time turned ideas into plans so that they can test and compare several solutions to solve this challenge. With industry guidance, PhD students William Roser and Yi-En Ji developed the plan sets, details, and design calculations for all the cold-formed steel walls. (Other components were detailed by industry partners.) Gradually, the working group participants donated nearly all the materials needed to build the assemblies, and one organization even helped build the facades using their apprenticeship program.
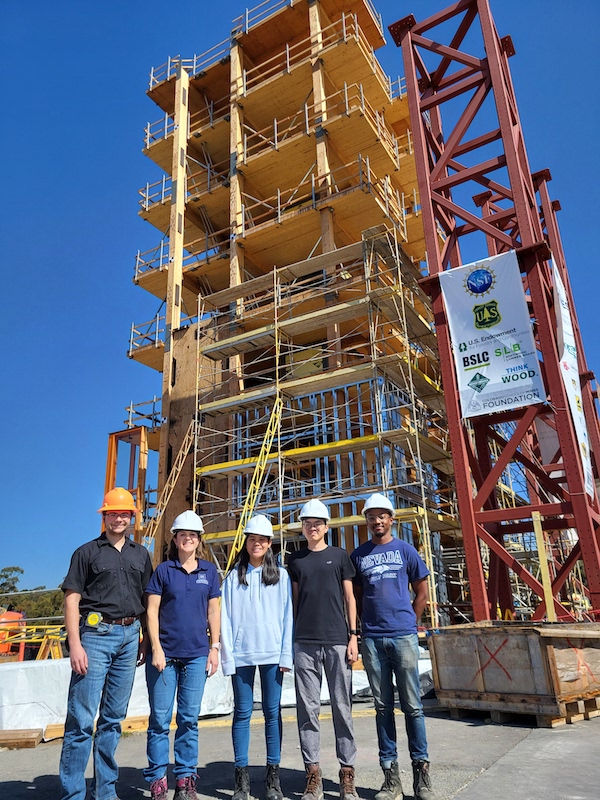
Ryan and her team (Roser, Ji and PhD student Sir Lathan Wynn) have been onsite since the fall supervising construction, preparing cables, installing sensors and cables, and performing numerous other tasks to get the building ready for testing. CEE Professor Mohamed Moustafa, along with postdoctoral researcher Luna Ngeljaratan and PhD student Mohammed Ibrahim will also make a noteworthy contribution to the project. They will use digital image correlation with cameras and targets to attempt to measure the movement of the building from base to top. Traditional drift sensors, string potentiometers attached to an off-table table sensor, don’t work well when the building is so tall.
The four-week test program is now getting underway on the world’s only outdoor shake table located at the UC San Diego's Englekirk Structural Engineering Center. The research program will carefully ramp up motions from very frequent (small) earthquakes to a very rare (maximum expected) earthquake, to develop a good understanding of how well the details hold up as the intensity of shaking is systematically increased.
The non-structural scope of work was also financially supported by U.S. Forest Service, Softwood Lumber Board, Computers and Structures, Inc, Allegion, and Exponent. Materials were donated by Construction Specialties, Allegion, Ehmcke Sheet Metal, CEMCO, USG, Simpson Strong-tie, Winco Window, Innotech Window and Door. The non-structural assemblies were installed by Southwest Carpenter’s Union, Ehmcke Sheet Metal, Long’s Glazing and Door, and Pacific Coast Drywall. Window airtightness testing is being conducted by RJC Engineers. The project could not be completed without all the generous contributions from industry.
The non-structural component scope of this project is sponsored by NSF Grant No. CMMI-1635363 and USFS Grant No. 19-DG-11046000-16. The use and operation of NHERI shake table facility is supported by NSF through Grant No. CMMI-2227407.Any opinions, findings and conclusions or recommendations expressed in this material are those of the author(s) and do not necessarily reflect the views of the National Science Foundation.